Antibiotic Resistance in Campylobacter
An outline of the molecular basis for antibiotic resistance in Campylobacter spp. by Declan Bolton and Séamus Fanning of Teagasc.Campylobacter infection is the most common cause of acute bacterial gastroenteritis in developed countries (Randall et al., 2003). After an average incubation period of three days, most cases present with abdominal pain and diarrhoea, and the infection is self-limiting. However, in approximately one-third of cases a period of fever and malaise accompanied by rigors, general aches, dizziness and delirium occurs for approximately 24 hours before the onset of gastrointestinal symptoms. Patients presenting with the latter tend to suffer more severe disease and require therapeutic intervention – usually a course of treatment with fluoroquinolone or macrolide antibiotics (O’Brien, 2002).
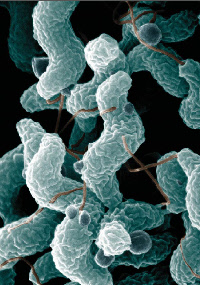
Image courtesy of Agricultural Research Service, United States Department of Agriculture. Photo by De Wood; digital colourisation by Chris Pooley.
Emerging Quinolone Resistance
In the last decade, antibiotic resistance in Campylobacter has emerged and spread rapidly. Many studies have attributed this resistance to the use of antimicrobial agents in poultry production (Humphrey et al., 2005). Control of the emergence and dissemination of antimicrobial resistance in Campylobacter requires a fundamental understanding of the molecular basis of the observed phenotypes, and several mechanisms for antibiotic resistance in Campylobacter have been identified, including modification (mutation) of target genes and activation of efflux pump systems.
Fluoroquinolones, such as enrofloxacin, target the DNA gyrase enzyme in Gram-negative bacteria. Resistance to this class of drug may develop when a mutation in the gyrA-encoding subunit of the DNA gyrase gives rise to one or more amino acid substitutions (Griggs et al., 2005) within the DNA-binding domain in a region termed the quinolone resistance-determining region (QRDR). For example, the Thr-86-Ile amino acid substitution in the QRDR is sufficient to confer a resistant phenotype in Campylobacter jejuni and Campylobacter coli (Piddock et al., 2003; Luo et al., 2003). Other modifications of the gyrA subunit have also been associated with quinolone resistance, including Asn-203-Ser (Piddock et al., 2003).
Mechanisms of Macrolide Resistance
Macrolides, such as erythromycin, inhibit protein synthesis in Campylobacter by binding to their ribosomes, causing dissociation of the peptidyl-tRNA and thereby preventing bacterial growth. Resistance is mediated through two mechanisms: modification of the antibiotic target by mutation; and, expulsion of the drug from the bacterial cell by efflux (Taylor et al., 2005).
The former is the most common mechanism. It may arise following point mutations at positions 2074 and/or 2075 in the 23S rRNA of the large (50S) ribosomal subunit or by mutations in the ribosomal proteins L4 and L22 (Taylor et al., 2005; Corcoran et al., 2006).
Other factors may also contribute to the resistance phenotype. Efflux of drug(s) confers a multi-drug resistance (MDR) phenotype to Campylobacter. The CmeABC efflux pump, for example, is known to promote both intrinsic and acquired resistance (Payot et al., 2004; Ge et al., 2005) to a range of antimicrobial agents, including fluoroquinolones and macrolides in Campylobacter species.
Resistance Research at Teagasc
Research was recently undertaken at Teagasc Food Research Centre, Ashtown, to investigate the role of both target gene mutations and efflux pump activity in fluoroquinolone and macrolide resistance in Campylobacter isolates from intensively reared Irish poultry.
Microdilution and E-test methods were initially applied to determine the minimum inhibitory concentrations (MIC) for two quinolone-based drugs (nalidixic acid and ciprofloxacin) and one macrolide (erythromycin). Using PCR and gene-sequencing technologies, the role of target gene mutations in resistant isolates was investigated, while the role of efflux was examined using phenylalanine arginine ß-naphthylamide (PAßN) – a known efflux pump inhibitor.
High-level resistance to nalidixic acid was attributed to amino acid substitutions Thr-86-Ile and Asn-203-Ser in gyrA of the DNA gyrase enzyme in some, but not all, isolates and, contrary to previous reports, the Thr-86-Ile substitution did not confer universal resistance to all quinolones. As expected, the 23S rRNA point mutation A2075G and/or amino acid substitutions in the L4 and/or L22 ribosomal proteins were identified in erythromycin-resistant strains. However, in contrast to previous studies, not all of the isolates carrying substitutions within the ß-hairpin region of the L22 ribosomal protein exhibited erythromycin resistance. In general, efflux did not contribute to either quinolone or macrolide resistance.
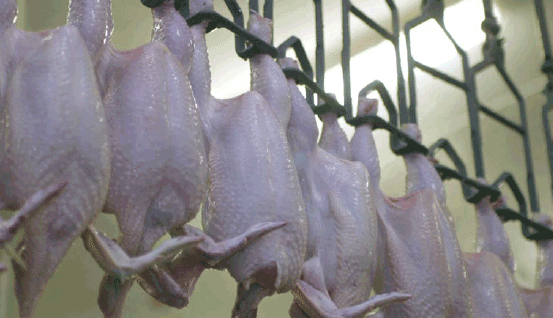
Relevance to Industry
Our research therefore supports the hypothesis that quinolone and macrolide antibiotic resistance in Campylobacter is mutation-based. While this trait may not be transferred horizontally from one organism to another, it is relatively stable in the Campylobacter population and can be passed vertically between parents and offspring cells.
The use of antibiotics during food production will confer a selective pressure, promoting the survival and proliferation of resistant strains, while susceptible organisms are eliminated from the microflora with inevitable adverse consequences for therapeutic intervention (in severe cases) and public health.
References
Corcoran, D., Quinn, T., Cotter, L. and Fanning, S. (2006). An investigation of the molecular mechanisms contributing to high-level erythromycin resistance in Campylobacter. International Journal of Antimicrobial Agents, 27: 40-45.
Ge, B., McDermott, P.F., White, D.G. and Meng, J. (2005). Role of efflux pumps and topoisomerase mutations in fluoroquinolone resistance in Campylobacter jejuni and Campylobacter coli. Antimicrobial Agents and Chemotherapy, 49: 3347-3354.
Griggs, D.J., Johnson, M.M., Frost, J.A., Humphrey, T., Jørgensen, F. and Piddock, L.J. (2005). Incidence and mechanism of ciprofloxacin resistance in Campylobacter spp. isolated from commercial poultry flocks in the United Kingdom before, during, and after fluoroquinolone treatment. Antimicrobial Agents and Chemotherapy, 49: 699-707.
Humphrey, T.J., Jørgensen, F., Frost, J.A., et al. (2005). Prevalence and subtypes of ciprofloxacin-resistant Campylobacter spp. in commercial poultry flocks before, during, and after treatment with fluoroquinolones. Antimicrobial Agents and Chemotherapy, 49: 690-698.
Luo, N., Sahin, O., Lin, J., Michel, L.O. and Zhang, Q. (2003). In vivo selection of Campylobacter isolates with high levels of fluoroquinolone resistance associated with gyrA mutations and the function of the CmeABC efflux pump. Antimicrobial Agents and Chemotherapy, 47: 390-394.
O’Brien S.J. The Campylobacter Sentinel Surveillance Scheme. (2000). Ciprofloxacin resistance in Campylobacter jejuni: case – case analysis as a tool for elucidating risks at home and abroad. Journal of Antimicrobial Chemotherapy, 50: 561-568.
Payot, S., Avrain, L., Magras, C., Praud, K., Cloeckaert, A. and Chaslus-Dancla, E. (2004). Relative contribution of target gene mutation and efflux to fluoroquinolone and erythromycin resistance, in French poultry and pig isolates of Campylobacter coli. International Journal of Antimicrobial Agents, 23: 468-472.
Piddock, L.J., Ricci, V., Pumbwe, L., Everett, M.J. and Griggs, D.J. (2003). Fluoroquinolone resistance in Campylobacter species from man and animals: detection of mutations in topoisomerase genes. Journal of Antimicrobial Chemotherapy, 5: 19-26.
Randall, L.P., Riddly, A.M., Cooles, S.W., et al. (2003). Prevalence of multiple antibiotic resistance in 443 Campylobacter spp. isolated from humans and animals. Journal of Antimicrobial Chemotherapy, 52: 507-510.
Taylor, D.E. and Tracz, D.M. (2005). Mechanisms of antimicrobial resistance in Campylobacter. In: Ketley, J.M. and Konkel, M.E. (Eds.): Campylobacter: molecular and cellular biology. Horizon Bioscience, Norfolk; p193-204.
December 2011