Vitamin Recommendations and Requirements for Turkeys
Dr Gilbert M. Weber of DSM Nutritional Products Ltd. in Basel, Switzerland offers practical recommendations for supplementing diets with vitamins to support the optimum health, performance and welfare of turkeys under commercial conditions.Summary
In order to entirely exploit the genetic potential of modern turkey breeds, an adequate supply with all nutrients including proteins, fats, carbohydrates, vitamins, minerals and water has to be secured. Among these elements vitamins are particularly important, since they are essential for optimum health as well as normal physiological functions of the birds. As most vitamins cannot be synthesized by poultry in sufficient amounts to meet physiological demand, they must be obtained from the diet. Insufficient supply of vitamins to high-performing turkeys for meat-production or breeding has detrimental effects on their productivity. This review summarizes the available scientific information, related to the requirements for and the beneficial effects of supplemental vitamins in turkeys. As there is good evidence that the minimum dietary vitamin levels, required to prevent clinical deficiencies, do not support optimum health, performance and welfare of turkeys under today’s commercial production conditions, more practical recommendations for dietary supplementation with vitamins are given.
Introduction
Vitamins are micronutrients, essential for life of man and animals. They are required for optimum health and normal physiological functions such as growth, maintenance or reproduction. As the poultry breeds of today are extremely productive, their vitamin nutrition needs particular attention in order to allow the birds to perform up to their genetic potential. While the need for added vitamins in animal nutrition is not disputed on principle, there are many questions related to the economic supplementation levels necessary to achieve optimum health and performance under production conditions. The aim of turkey nutrition is to maximize performance in fattening poults and to optimize egg production, fertility and hatchability in breeding birds. Vitamins are vitally important in all aspects of poultry nutrition not only for the physiological needs of the growing poult and the breeding hen, but also for the embryonic development and the viability of the chicks. This paper is intended to provide information related to the specific needs of turkeys for growth and health, the formation of the skeleton, fertility of males and females as well as embryonic development and hatchability.
Vitamin Requirements for Preventing Deficiency and Improving Performance
The first determination of vitamin A requirements of turkeys resulted in a minimum dietary level of 5,000 IU/kg feed, needed to prevent gross signs of avitaminosis A. But the authors mentioned already that higher levels might be necessary to prevent microscopic vitamin A deficiency lesions as well (Van Reen et al., 1951). Based on optimum growth and vitamin A liver stores of turkey poults, at least 4,721 IU vitamin A per kg were found to be necessary in a later study (Couch et al., 1971). Jeroch et al. (1978) concluded that the vitamin A requirement of the fattening turkey was met with 3,000 IU/kg feed. Later the same group found that 2,000 IU vitamin A would satisfy the basic requirements for growth, but that 5,000 IU/kg were necessary to fill the liver storage compartment. A 20-day depletion period on low vitamin A supply resulted in vitamin A deficiency symptoms after 1 week only (Prinz et al., 1981). More recently, 11,000 IU vitamin A per kg were found to be necessary to avoid epithelial tissue damage (white plaques in the oropharynx and esophagus) in turkeys. Within only 3 weeks on a vitamin A deficient diet liver vitamin A levels were reduced and squamous metaplasia developed (Aye et al., 2000a). In a commercial flock, vitamin A deficiency of turkey poults did increase the daily mortality by 3% and induced the classical clinical picture of disorders, being stunted growth and ruffled feathers, incoordination of movements and general depression (Cortes et al., 2006). Excess vitamin A significantly depressed growth and above 600 IU/g body weight resulted in death of the birds. Inflammatory lesions around the eyes and mouth occurred and bone ash was significantly reduced (Veltmann and Jensen, 1986). Beta-carotene (BC) can also be used to supply vitamin A to turkey poults. Body weight and food intake increased with dietary BC supplementation. Plasma and liver BC and liver retinol increased with dietary BC, but plasma retinol was not affected (Stevens and Salmon, 1989).
The clinical appearance of vitamin D3 deficiency in turkeys has been investigated predominantly in field studies. In two cases of field rickets of turkey poults the primary observation was lameness and the sole necropsy finding of significance was hypomineralization of bone. This diagnosis was confirmed radiographically; thin cortices and wide growth plates were noted (Walser et al., 1980). In several clinical cases of rickets the mean plasma calcium concentration in rachitic poults was not significantly different from that of controls. But mean plasma phosphorus concentrations were lower and values for alkaline phosphatase activity were greater in the rachitic birds (Olson et al., 1981). Vitamin D3-deficient poults were found to be lame and to have significant decreases in weight gain and in longitudinal skeletal growth. Therapeutic supplementation of vitamin D3 via the drinking water did not alleviate all these changes, but calcium and 25-hydroxyvitamin D3 concentrations were significantly improved (Perry et al., 1991). In terms of requirements, in a rather old study, the vitamin D3 necessary for optimizing growth of turkeys was found to be 1,000 and 500 IU/kg for starter feed and fattening diets, respectively (Jeroch et al., 1978). Poults on vitamin Ddeficient diets became rachitic within 17-24 days. Supplementation of the diet with 12.5 micrograms cholecalciferol per kg was sufficient to promote maximal body weight and normal plasma calcium, plasma phosphorus and bone ash. Feeding vitamin D-deficient rachitic birds for 4 days a diet containing 50 micrograms cholecalciferol per kg restored plasma calcium as well as phosphorus and bone ash, but did not increase body weight to the level of the controls (Bar et al., 1982). Vitamin D3 included at 2,400 IU/kg feed increased body weights of poults significantly and lowered plasma alkaline phosphatase, compared to birds on a diet containing 900 IU/kg. Tibia ash was significantly greater with the higher vitamin D3 supplementation (Stevens et al., 1983). When increasing the level of vitamin D3 in the hen diet, poult weight at hatching and during fattening was improved and mortality was reduced. Bone ash and breaking strength increased and the severity of rickets score decreased (Stevens et al., 1984b). An antagonistic interaction between vitamins A and D was observed in male turkey poults fed a diet containing the required level of vitamin D and a high level of vitamin A. This combination resulted in severe lameness, growth depression and a rachitic-like condition (Metz et al., 1985).
Turkey poults, fed a vitamin E deficient diet abruptly developed neurologic signs such as tremor, incoordination and recumbency. Associated lesions included recent ischemic necrosis of the cerebellum and the spinal cord, resembling nutritional encephalomalacia of chicks (Jortner et al., 1985). On a low vitamin E diet both liver and serum alpha-tocopherol decreased markedly in turkeys from 1 to 14 days of age. Supplementation with 200 mg/kg vitamin E could only partly alleviate the reduction of alpha-tocopherol in liver and serum (Soto-Salanova et al., 1993). Likewise feeding turkey poults with 150 ppm vitamin E increased the concentrations of alpha-tocopherol in the liver and plasma, but did not completely overcome the decrease in liver and plasma alpha-tocopherol. Although the 150 ppm vitamin E diet had no effect on poult body weight and feed efficiency, it decreased the susceptibility of liver and red blood cells to in vitro peroxidation (Applegate and Sell, 1996). Supplementation of turkeys with 50 mg vitamin E per kg strongly reduced TBARS (thiobarbituric acid reactive substances) in the liver, indicating antioxidative protection of this tissue, which could not be observed with increasing levels of selenium (Mueller et al., 2009). Since field observations have shown that vitamin E status of poults is often inadequate during the post-hatching period, subtle adverse effects on health and metabolic efficiency are probable. Supplementation of poults with 100 to 150 mg/kg of vitamin E in the first diet is therefore advisable (Sell, 1996). However, when the turkeys were free of disease, satisfactory performance and well-being of male turkeys during the fattening period with 20 mg vitamin E per kg diet seemed to be possible (Sell et al., 1997). At equivalent feeding levels of vitamin E, plasma and liver tocopherol concentrations were found to be 1.5- to 4.5-fold lower in turkeys than in chicks (Sklan et al., 1982). Likewise, the resultant concentration of alphatocopherol in the yolk of the chicken egg was found to be four to five times greater than that observed for turkeys, resulting in lower vitamin E levels in the livers of the hatchlings (Surai et al., 1998).
Dietary vitamin K1 was demonstrated to be efficacious in reducing plasma prothrombin time, but had little, if any, effect on bone development in 1- to 14-d-old turkeys (Jin et al., 2001).
Except for biotin the scientific information related to B-vitamin requirements for turkeys is limited. Polyneuritis was described as a clinical deficiency sign for vitamin B1, which could be prevented by injection or dietary supplementation of thiamine (Charles et al., 1972). Erythrocyte counts of turkey hens, deficient in vitamin B2 (riboflavin), vitamin B6 (pyridoxine) or pantothenic acid was described to be markedly below that of hens on a complete diet (Whiteside et al., 1962). Jeroch et al. (1978) reported that the requirements for vitamins B2, B6, B12, niacin, pantothenic acid, folic acid and biotin of the fattening turkey were covered by the respective amounts contained in the ration components. For folic acid, the requirement for breeder hens and their progeny was determined as 1.23 and 0.81 mg/kg feed, respectively (Miller and Balloun, 1967).
Dietary levels between 231 and 284 mcg/kg biotin were found to be adequate for obtaining maximum growth and to reduce leg weakness in turkey poults (Jensen and Martinson, 1969). In a later study, an addition of 220 mcg biotin per kg feed was necessary to avoid deficiency symptoms and to increase pyruvate carboxylase activity, but this supplementation level failed to improve growth rate of turkeys (Atwal et al., 1972). In a feeding trial with male poults on a diet with animal protein a supplementation with biotin gave excellent performance responses. A highly significant improvement in growth was obtained and feed efficiency was found to be closely related to growth rate (Krueger et al., 1976). Maximum activity of blood pyruvate carboxylase activity in turkeys was related positively to the added biotin level and optimum activity was attained with supplemental levels above those required to give maximal growth response (Whitehead and Bannister, 1978). In a complex experimental design the bioavailability of biotin from different protein supplements and cereal grains for turkey poults was determined and found to be low for triticale, wheat, barley and sorghum, moderate for canola and soybean meal and high for corn (Misir and Blair, 1988).
Although, vitamin C can be synthesized by poultry, supplementation has shown beneficial effects in broilers and laying hens. In fattening turkey poults, however, no respective studies have been published. For this and the other vitamins, for which scientific information on the requirement for turkeys is lacking, the needed vitamin supplementation levels have to be extrapolated from studies with broiler chickens.
Vitamin Requirements for Optimizing Health
Vitamin A was demonstrated to improve disease resistance in turkeys, presumably via a better immune response. When poults were immunized with Newcastle disease virus (NDV) and turkey pox vaccines, the increasing dietary concentrations of vitamin A enhanced the proliferative response and the antibody titres to NDV and turkey pox (Sklan et al., 1995). Although the time course of the systemic spread of Pasteurella multocida infection in turkeys was not changed, the increased mortality, seen in the vitamin Adeficient turkeys, was associated with immune system impairment (Aye et al., 2000b).
Low supplementation levels of vitamin A increased the incidence of candidiasis in turkeys. Poults on a vitamin A deficient diet developed more severe crop lesions and were shedding significantly more Candida albicans cells in their feces than birds on a vitamin A adequate diet (Tripathy et al., 1967). Male turkeys, fed a high vitamin A diet recovered faster from a coccidiosis infection than those on low vitamin A (Mitrovic and Schildknecht, 1973). Eimeria meleagrimitis infection markedly decreased plasma retinol levels, resulting in a strong reduction of the concentration of retinol in liver. In contrast, Eimeria adenoeides infection caused little change in either plasma or liver retinol levels (Augustine and Ruff, 1983). Increasing vitamin A supplementation tended to decrease histomoniasis mortality and morbidity (Whitmore et al., 1968).
In an experimental model with turkey osteomyelitis complex (TOC) vitamin D3 supplementation protected the poults from the immunosuppressive effects of multiple injections with dexamethasone, emphasizing the important role of vitamin D3 for health and disease resistance (Huff et al., 2000). In a subsequent study there was a tendency of lower mortality, lower TOC scores and lower percentage of TOC in the treatment with the vitamin D3 metabolite 25-hydroxyvitamin D3 (Huff et al., 2002). The supplementation of 25-(OH)D3 as a source of vitamin D3 in turkey rations was shown to be safe up to a level of 10x (990 mcg/kg) of the recommended dietary dose level as assessed by growth and general health parameters (Lanenga et al., 1999).
Vitamin E supplementation in excess of requirement significantly increased humoral immune response or disease resistance. Turkeys, supplemented with high vitamin E and infected with E. coli, had reduced mortality and increased HA titers (Nockels, 1979). But in a challenge experiment, an addition of 300 mg vitamin E per kg to the diet of turkeys did not alleviate the adverse effects of E. coli infection in young turkeys (Sell et al., 1997b). And concentrations of circulating antibodies against Newcastle disease and turkey pox vaccines were negatively influenced by dietary vitamin E, indicating that humoral immune responses might be affected by excessive vitamin E (Friedman et al., 1998). In contrast to these findings, turkeys, challenged with an air sac inoculation of E. coli, and treated with vitamin E via the drinking water, had significantly lower mortality and air sacculitis scores and the isolation rate of E. coli from the liver was decreased, indicating that treatment of turkey poults with vitamin E can reduce disease incidence and mortality from colisepticemia (Huff et al., 2004). In turkeys with 400 ppm supplemental vitamin E Heffels-Redmann et al. (2001) did not observe a difference in the humoral immune response to vaccinal B-cell dependent Newcastle disease, when compared to an unsupplemented control, but reported an improvement of the cellular immune response measured in the lymphocyte transformation assay using concavalin A as mitogen. Furthermore, significantly higher body weights were found in the vitamin E group. In turkeys, supplemented with graded levels of vitamin E and orally inoculated with Listeria monocytogenes CD4+ and CD8+ peripheral blood T lymphocytes were elevated in poults given 200 mg/kg vitamin E. Furthermore, L. monocytogenes was cultured less frequently from cloacal swabs of the vitamin E-treated group, when compared to the unsupplemented controls (Zhu et al., 2003).
A syndrome, characterized by hock disorder, broken feathers, dermatitis and diarrhea was observed in turkey poults, of which both incidence and severity could be markedly reduced by supplementation with biotin. Feeding of folic acid and calcium pantothenate had no effect, but together with biotin poults essentially free of the disorder could be produced (Robblee and Clandinin, 1970).
Severe pododermatitis is a common lesion in turkeys. Both dietary factors and poor litter conditions are documented etiologies of footpad lesions. Turkey poults on a semipurified diet low in biotin developed severe dermatitis of the footpad. A supplementation between 275 (females) and 325 mcg/kg (males) was necessary to prevent the appearance of these deficiency symptoms (Dobson, 1970). This problem might be related to the physiology of the skin. In biotin deficient turkeys a high concentration of very long chain fatty acids was found in the neutral lipid fraction of the skin, which were completely absent in biotin supplemented birds. Further, almost onethird of the fatty acids of diester waxes in biotin deficient birds were unsaturated, while those from biotin supplemented birds were predominantly saturated (Logani et al., 1977). While the severity of foot pad dermatitis in poults maintained on dry litter was decreased by the addition of biotin, this vitamin did not influence the severity of the lesions when poults were maintained on wet litter (Harms and Simpson, 1977). Later, research of Clark et al. (2002) has clearly demonstrated the association between elevated biotin levels and reduced pododermatitis. However, in a recent study it was found that higher supplements of biotin than those currently used in the industry did not prevent the occurrence of footpad dermatitis in poults (Mayne et al., 2007). Another biotin-dependent disorder was described by Whitehead and Siller (1983). Turkeys fed on diets of low biotin content developed clinical signs of fatty liver and kidney syndrome and on autopsy showed pale and swollen livers and kidneys. Affected birds suffered from severe hypoglycaemia and hepatic glycogen was depleted.
Vitamin Supplementation and Meat Quality
There is an impressive body of scientific literature, documenting the powerful effect of high levels of dietary vitamin E on the reduction of lipid oxidation in the post-mortem muscle of various farm animals. In turkeys supplementation with vitamin E significantly reduced TBARS (thiobarbituric acid reactive substances) numbers in both raw and cooked burgers during refrigerated and frozen storage, which indicates a protection of the meat against oxidative rancidity by dietary vitamin E (Wen et al., 1996). Excess dietary iron did not adversely affect the oxidative stability of thigh meat of turkey, but stability was reduced by injected Fe. Dietary vitamin E, at a level of 150 mg/kg, consistently reduced oxidative deterioration of the meat (Bartov and Kanner, 1996). Turkey toms were reared on diets, containing graded vitamin E levels. The TBARS values in breast meat after refrigeration or frozen storage were inversely related to the dietary vitamin E levels. Furthermore, high vitamin E supplementation produced the most typical and acceptable turkey meat flavors with the fewest oxidized off-flavor notes (Sheldon et al., 1997). Mean alpha-tocopherol levels in breast and leg muscle from turkeys were significantly higher in the elevated vitamin E groups compared to the controls. In both leg and breast muscles lipid oxidation was suppressed by vitamin E supplementation (Higgins et al., 1998b). Also in raw overwrapped and vacuum-packaged turkey leg and breast patties vitamin E supplementation of turkeys during rearing significantly reduced TBARS numbers (Higgins et al., 1998a). After cooking the development of warmed-over flavor was reduced in meat patties from vitamin E turkeys (Higgins et al., 1999). In addition to delaying lipid oxidation in the meat of vitamin E supplemented poults during refrigerated storage also color stability was improved (Mercier et al., 1998). Although higher supplementation of poults with vitamin A decreased plasma tocopherol, the concomitant supplementation of high dietary vitamin A and E was most efficacious to reduce lipid oxidation in turkey meat (Sallmann et al., 1998).
Mineral Metabolism and Skeletal Development
The formidable progress in the genetic set-up of high performing turkey breeds has developed poults with fast growth and improved breast yield, but often being accompanied by an increased susceptibility to skeletal abnormalities. Currently, the turkey industry is challenged by leg problems, especially in the production of heavy turkeys. It is well established that lameness reduces performance, increases susceptibility to disease and is considered to be a serious welfare concern. More than in growing poults, the development of a strong skeletal frame is of utmost importance for breeding turkeys, since maximizing bone mass before the onset of lay prevents the pullets from a fatal demineralization of the bones, which typically reduces longevity and productivity of the birds. A considerable vitamin D activity is required to support an adequate skeletal development and to avoid leg problems or osteoporosis. For specific conditions, in which the utilization of the dietary vitamin D3 is compromised, 25-hydroxyvitamin D3 (25- (OH)D3; Hy•D®), the first metabolite in the cascade of vitamin D mobilization, was developed for the poultry industry. 25-(OH)D3 has been demonstrated to support the homeostatic function of vitamin D3, which is important to provide sufficient minerals for incorporation into the bone matrix and to avoid bone disorders of various origins (Soares et al., 1995). A study to determine the effects of 25-(OH)D3 on performance and bone mineralization in turkeys showed that poults fed 40 to 100 micrograms (mcg) 25- (OH)D3/kg of feed gained more weight and had stronger bones than the control birds on vitamin D3 at a level of 4’750 IU/kg (Owens et al., 2000). Furthermore, when feeding 25- (OH)D3 to layers and breeders, more eggs were produced and the stability of the eggshell was improved (Soares et al., 1995). For vitamin D3, supplemented above established requirements to turkey hens, no effects on egg production or hatchability of fertile eggs was observed, indicating that the D3-metabolite 25-(OH)D3 has superior physiological functions than its mother compound (Menge et al., 1977).
Fertility of Male and Female Turkeys
Testes growth was delayed in turkey toms fed low vitamin A diets, but there were no significant differences in sperm volume or sperm concentration as a result of dietary treatments (Miller et al., 1977). Fertility problems, induced in male poultry by a high poly-unsaturated fatty acids (PUFA) content of the diet could be counteracted with high vitamin E supplementation. In a long term trial, Arscott and Parker (1967) confirmed the adverse effect of a high linoleic acid – low vitamin E diet on semen concentration, but demonstrated that the addition of vitamin E to such birds restored fertility and improved semen concentration to the level obtained from males receiving vitamin E throughout the trial. Similar results were achieved when using fish oil in combination with vitamin E (Zanboni et al., 2006). The sperm content of alpha-tocopherol was more than doubled, when supplying 120 mg/kg vitamin E to turkey males compared with 60 mg/kg in the controls, resulting in spermatozoa being less susceptible to in vitro induced oxidation. This effect is likely due to the powerful antioxidant activity of alpha-tocopherol, being able to quench oxygen radicals in fat-soluble matrices. Under aerobic conditions, which are required to maintain the viability of turkey sperm, excess oxygen during in vitro storage resulted in lipid peroxidation, causing membrane damage, reduced sperm motility and subsequent fertility. Donoghue and Donoghue (1997) found that the addition of vitamin E to extended turkey semen improved sperm survival, membrane integrity and reduced the loss of motility after 48 h storage. Since artificial insemination with stored semen with higher malon-dialdehyde (MDA) values yielded lower fertility rates, lipid peroxidation must be considered a significant factor, affecting the fertility of stored turkey sperm. Exogenous vitamin E, added to turkey semen, failed to reduce lipid peroxidation during liquid storage, although MDA values increased up to three-fold (Long and Kramer, 2003). This finding might indicate that for the prevention or reduction of lipid peroxidation in semen liquid, a dietary supplementation of vitamin E at supranutritional levels might be more efficacious, since the physiological deposition of sufficient alpha-tocopherol in the sperm membranes could protect these structures from oxidative damage.
Multinucleated giant cells (MCG) in the testes of male turkey breeders are an indication of tissue degeneration and might negatively impact on their fertility. Dietary vitamin C did not affect semen traits as such, but entirely suppressed the appearance of MCG, probably due to the anti-oxidative activity of ascorbic acid, which was supposed to delay such degenerative developments in the male reproductive organs (Neuman et al., 2002).
Related to female fertility, a rather old publication reported that the addition of supplemental vitamin E to the ration of female turkeys had no apparent effect on either fertility or hatchability of eggs (Leighton et al., 1968). Later, a requirement of 20 mg vitamin E per kg feed for breeding hens was postulated, provided that the diet contained sufficient selenium and was not rich in PUFAs (Hennig et al., 1986). A close relation between the vitamin E content of the feed and that of the yolk was found. A supplement of 7 or 14% of vitamin E-free sunflower oil decreased hatchability of fertile eggs as the vitamin E content of the yolk sac was found to be drastically reduced. Consequently, it was suggested to use sunflower oil rich in vitamin E or to add supplemental vitamin E to the diet of breeding hens in order not to compromise on hatchability of the embryos. When being fed to laying hens, vicine, a compound occurring in faba beans, had very dramatic effects, i.e. it depressed feed consumption, egg weight, fertility and hatchability of eggs, packed cell volume and erythrocyte hemoglobin levels and led to increased liver problems. Muduuli et al. (1982) demonstrated that supplementation of laying hens with vitamin E slightly increased egg weights, markedly improved fertility as well as hatchability of eggs and lowered liver weights and lipid levels. It was concluded that vicine or its metabolites or both caused peroxidation of cellular components, which resulted in abnormal lipid transport or synthesis, increased fragility of erythrocytes, and reduced fertility. These effects could be overcome to varying extents by supplemental vitamin E.
Embryonic Development and Hatchability
It has been known for some time that vitamin D is essential for the hatchability of poultry eggs. A deficiency of this vitamin results in embryos having a shortened mandible and other skeletal deformities with death occurring after 18 days of incubation (Kubota et al., 1981). Avian embryos assimilate large amounts of calcium (Ca) in their bones in a short amount of time and because yolk contains insufficient amounts of Ca, this mineral must be derived from the eggshell. In turkeys it was demonstrated that hens receiving low levels of vitamin D3 produced fewer eggs, which were lighter in weight and had thinner shells than those laid by hens receiving the higher levels of vitamin D3. Fertility was not affected by treatment; however, hatchability of low-D3 eggs was reduced by 48% (Stevens et al., 1984a). High dietary vitamin D3 (4,000 IU/kg) for breeder hens did also positively influence chick quality, resulting in better body weight gains and higher tibia ash after hatch (Atencio et al., 2005). The active hormonal form of vitamin D3 (1,25-(OH)2D3) is not transferred into the egg and thus cannot support hatchability in vitamin D deficient hen diets (Sunde et al., 1978). However, the first D3- metabolite 25-(OH)D3 is easily deposited into the turkey egg and is then converted to 1,25-(OH)2D3 by the embryonic kidney after day 8 of embryonation to support the transfer of Ca from the eggshell and assimilation into the embryonic skeleton. Accordingly, Manley et al. (1978) observed a significant improvement in hatchability of fertile turkey eggs, when 25-(OH)D3 was added to the diet as compared to vitamin D3.
Within the group of the water-soluble vitamins, biotin has been the subject of many investigations. Back in 1976, Bradley et al. observed that a supplementation of Leghorntype breeder hens with biotin at a level of 550 micrograms (mcg)/kg of diet improved productivity and feed efficiency. Biotin supplementation had no effect on egg size or adult livability, but fertility and hatchability were both significantly improved. Increased hatchability was due to a decreased number of early dead, late dead and pipped embryos that failed to hatch. Likewise, an improvement of egg production as well as hatchability was observed, when a low-protein diet was fortified with biotin. Furthermore, poults hatched from eggs of supplemented breeder hens showed an improvement in growth rate (Atkinson et al., 1976). The value of supplemental biotin for increasing hatchability in turkey hens was determined in the last two-thirds of the production cycle (Robel, 1991). Eggs from hens fed 623 mcg/kg had the fewest embryonic deaths during incubation. Concentrations of biotin in egg albumen increased with incremental dietary biotin levels, but egg yolk concentrations were stable. About 38 mcg of biotin per egg produced highest embryo survival. The biotin demand for hatchability increased up to a level of 800 mcg/kg with maternal age. In a similar experiment a corn-soybean meal-based breeder diet was supplemented from the time of photo-stimulation with biotin to provide a low (178 mcg/kg) and a high (750 mcg/kg) dietary level of this vitamin (Chen et al. 1994). Egg production and hatchability of fertile eggs from hens fed the high level of biotin was significantly greater during 50 to 54 weeks of age and were improved by 22% (egg production) and 10% (hatchability), respectively. The higher level of dietary biotin seemed particularly beneficial in supporting later reproductive performance in this study.
The value of dietary vitamin B6 (pyridoxine) supplementation for increasing hatchability was determined in Large White turkey hens, kept on a basal corn and soybean meal diet (Robel, 1992). The hens were photo-stimulated at 31 weeks of age and two weeks later were assigned to dietary treatments, containing 0, 6, 12 and 18 mg of supplemented pyridoxine/kg of diet. Although the vitamin B6 concentration in egg yolk was stable, its concentrations in egg albumen increased with incremental dietary pyridoxine levels. However, the average concentration of vitamin B6 in egg albumen was only 4% of the average level in egg yolk. Incremental dietary doses of supplemented pyridoxine above the basal diet level did not result in increasing hatchability or egg vitamin B6 levels. Since direct injection of pyridoxine into fertile eggs resulted in a significant improvement of hatchability (Robel and Christensen, 1991), either the dietary supplementation with vitamin B6 was too low or the deposition of dietary pyridoxine was inadequate.
A possible interaction between the requirements for riboflavin and vitamin B12 for hatchability was investigated in White Leghorn hens (Tuite and Austic, 1974). The vitamin B12 requirement was found to be 3.60 mcg/kg of diet and that for dietary riboflavin was approximately 2.75 mg/kg. Dietary riboflavin at deficient or practical levels was found not to affect the vitamin B12 concentration in egg yolk or the vitamin B12 requirement for optimum hatchability, while high levels of vitamin B12 exerted a striking negative effect on riboflavin egg yolk concentrations. The high B12 supplementation levels, however, increased egg size. Dietary vitamin B12 in breeding hens was also demonstrated to counteract the negative effect of increasing protein levels on hatchability (Patel and McGinnis, 1977). Furthermore the chicks, hatched from eggs of hens on a medium protein level and adequate vitamin B12 showed better early growth and livability.
The need for niacin supplementation was investigated in turkey hens fed a cornsoybean meal diet with an intrinsic niacin contribution of 23.6 mg/kg diet (Harms et al., 1988). No supplemental niacin was required for maximum egg production, fertility or hatchability. However, body and egg weights were significantly increased when 8.4 and 16.7 mg niacin were added per kg of diet, respectively. These data indicate that supplemental niacin was required for maximizing egg weight of turkey breeders.
Three supplementation levels of pantothenic acid were given to a commercial strain of turkeys by Robel (1993). Incremental dietary pantothenic acid levels increased the transfer of pantothenic acid in eggs, but did not result in a hatchability increase over the unsupplemented pantothenic acid basal diet.
The results of a similar study with folic acid showed that incremental dietary supplemental folic acid levels produced a positive linear response pattern on the transfer of folic acid in eggs, but did not result in a hatchability increase over the unsupplemented folic acid basal diet (Robel, 1993). Hatchability increase did neither occur for eggs injected with folic acid. However, egg and poult weights were significantly increased in eggs, containing higher levels of folic acid.
Although birds are capable to synthesize vitamin C, supplemental ascorbic acid has been demonstrated to beneficially influence egg reproduction in laying hens, particularly under tropical conditions (Panda et al., 2008). A supplementation of broiler breeders with 75 mg ascorbic acid/kg diet did however not influence their egg production, egg weight, eggshell porosity, fertility, hatchability or plasma ascorbic acid (Creel et al., 2001).
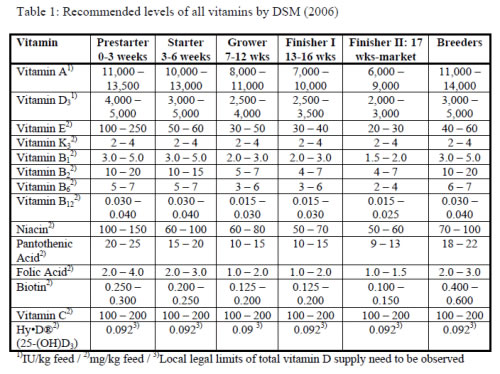
Conclusions
It is now well recognized by the feed industry that the minimum dietary vitamin levels, required to prevent clinical deficiencies may not support optimum health, performance and welfare of poultry. The predominant reason for that is the rapid progress in genetic development of the breeds, having resulted in impressive improvements of productivity, which considerably increased the demand for vitamins. Furthermore, the intensive turkey husbandry conditions for meat production generates a certain level of metabolic, social, environmental and disease stresses, causing a higher susceptibility to vitamin insufficiencies. But also the breeding birds, which have to be supplied with vitamins not only for their own maintenance, but also for deposition into the egg to support the development of the embryo, merit special attention. The available literature on effects of supplemental vitamins to turkeys, however, is limited and partly outdated. Nutritionists may therefore consult the recommendations from breeding companies or vitamin suppliers (table 1), which are based on both research and experience from industry practice. These supplementation levels generally exceed the minimum requirements, postulated by governmental institutions, since they aim at supporting optimum health and performance of domestic animals in the most cost-effective way.
References
Applegate, T.J. and J.L. Sell (1996): Poult. Sci., 75: 881-890.Arscott, G.H. and J.E. Parker (1967): J. Nutr., 91:219-222.
Atencio, A., H.M. Edwards Jr. and G. Pesti (2005): Poult. Sci., 84: 1058-1068 (2005).
Atkinson, R.L., K.K Krueger, J.W. Bradley, J.R. Couch and W.F. Krueger (1976): Nutr. Reports Internat. 13: 225-235.
Atwal, A.S., A.R. Robblee and L.P. Milligan (1972): Poult. Sci., 51: 2074-2077.
Augustine, P.C. and M.D. Ruff (1983): Avian Dis., 27: 963-971.
Aye, P.P., T.Y. Morishita, Y.M. Saif, J.D. Latshaw, B.S. Harr and F.B. Cihla (2000a): Avian Dis., 44: 809-817.
Aye, P.P., T.Y. Morishita, Y.M. Saif and M. Jonas (2000b): Avian Dis., 44: 818-826.
Bar, A., S. Edelstein, U. Eisner, I. Ben-Gal and S. Hurwitz (1982): J. Nutr., 112: 1779- 1786.
Bartov, I. and J. Kanner (1996): Poult. Sci., 75: 1039-1046.
Bradley, J.W., R.L. Atkinson and, W.F. Krueger (1976): Poult. Sci., 55: 2490-2492.
Charles, O.W., D.A. Roland and H.M. Edwards Jr. (1972): Poult. Sci., 51: 419-423.
Chen, F., S.L. Noll and P.E. Waibel (1994): Poult. Sci., 73: 682-686.
Clark, S., G. Hansen, P. McLean, P. Bond Jr., W. Wakeman, R. Meadows and S. Buda (2002): Avian. Dis., 46: 1038-1044.
Cortes, P.L., A.K. Tiwary, B. Puschner, R.M. Crespo, R.P. Chin, M. Bland and H.L. Shivaprasad (2006): J. Vet. Diagn. Invest., 18: 489-494.
Couch, J.R., C.R. Creger and R. Chavez (1971): Br. Poult. Sci., 12: 367-371.
Creel, L.H., D.V. Maurice, S.F. Lightsey and L.W. Grimes (2001): Br. Poult. Sci., 42: 96-101.
Dobson, D.C. (1970): Poult. Sci., 49: 546-553.
Donoghue, A.M. and D.J. Donoghue (1997): Poult. Sci., 76: 1440-1445.
DSM (2006): DSM Vitamin supplementation guidelines.
Friedman, A., I. Bartov and D. Sklan (1998): Poult. Sci., 77: 956-962.
Harms, R.H. and C.F. Simpson (1977): Poult. Sci., 56: 2009-2012.
Harms, R.H., N. Ruiz, R.E. Buresh and H.R. Wilson (1988): Poult. Sci., 67: 336-338.
Heffels-Redmann, T. Redmann and K. Voelckel (2001): Proc. Vitamine und Zusatzstoffe in der Ernährung von Mensch und Tier, pp 101-106.
Hennig, A., E. Marckwardt and G. Richter (1986): Arch Tierernähr., 36: 519-529.
Higgins, F.M., J.P. Kerry, D.J. Buckley and P.A. Morrissey (1998a): Br. Poult. Sci., 39: 596-600.
Higgins, F.M., J.P Kerry, D.J. Buckley, and P.A Morrissey (1998b): Meat Sci., 50: 373- 383.
Higgins, F.M., J.P. Kerry, D.J. Buckley and P.A. Morrissey (1999): Br. Poult. Sci., 40: 59-64.
Huff, G.R., W.E. Huff, J.M. Balog and N.C. Rath (2000): Poult. Sci., 79: 672-679.
Huff, G.R., W.E. Huff, J.M. Balog, N.C. Rath, H. Xie and R.L. Horst (2002): Poult. Sci., 81: 958-965.
Huff, G.R., W.E. Huff, J.M. Balog, N.C. Rath and R.S. Izard (2004): Avian Dis., 48: 324-331.
Jensen, L.S. and R. Martinson (1969): Poult. Sci., 48: 222-230.
Jeroch, H., M. Prinz and A. Hennig (1978): Arch. Tierernähr., 28: 53-65.
Jin, S., J.L. Sell and J.S. Haynes (2001): Poult. Sci., 80: 607-614.
Jortner, B.S., J.B. Meldrum, C.H. Domermuth and L.M. Potter (1985): Avian Dis., 29: 488-498.
Krueger, K.K., R.L. Atkinson, J.R. Couch and W.F. Krueger (1976): Poult. Sci., 55: 497- 501.
Kubota, M., E. Abe, T. Shinki and T. Suda (1981): Biochem. J., 194: 103-109.
Lanenga, M., M. Terry, J.L. Mc Naughton and L.E. Stark (1999): Vet. Human Toxicol., 41: 75-78.
Leighton, A.T. Jr., I.F. Carte, L.M. Potter, J.W. Coleman and W.L. Beane (1968): Poult. Sci., 47: 1797-1806.
Logani, M.K., D.B. Nhari, P.D. Forbes and R.E. Davies (1977): Lipids, 12: 626-628.
Long, J.A. and M. Kramer (2003): Poult. Sci., 82: 1802-1807.
Manley, J.M., R.A. Voitle and R.H. Harms (1978): Poultry Sci. 57: 290-292.
Mayne, R.K., R.W. Else and P.M. Hocking (2007): Br. Poult. Sci., 48: 291-298.
Menge, H., E.G. Geis, P.E. James and L.T. Frobish (1977): Poult. Sci., 56: 1472-1480.
Mercier, Y., P. Gatellier, M. Viau, H. Remignon and M. Renerre (1998): Meat Sci., 48: 301-318.
Metz, A.L., M.M. Walser and W.G. Olson (1985): J. Nutr., 115: 929-935.
Miller, D.L. and S.L. Balloun (1967): Poult. Sci., 46: 1502-1508.
Miller, D.H., J.W. Bradley and T.M. Ferguson (1977): Poult. Sci., 56: 1086-1091.
Misir, R. and R. Blair (1988): Poult. Sci., 67: 1274-1280.
Mitrovic, M. & E.G. Schildknecht (1973): Poult. Sci., 52: 1253-1260.
Muduuli, D.S., R.R. Marquardt and W. Guenter (1982): Br. J. Nutr., 47: 53-60.
Mueller, A.S., J. Fischer, E. Most and J. Pallauf (2009): J. Anim. Physiol. Anim Nutr., 93: 313-324.
Neuman, S.L., J.I. Orban, T.L. Lin, M.A. Latour and P.Y. Hester (2002): Poult Sci., 81: 265-268.
Nockels, C.F. (1979): Fed. Proc., 38: 2134-2138.
Olson, W.G., H.E. Dziuk, M.M. Walser, G.F. Hanlon, P.E. Waibel, J.B. Stevens, N.A. Jorgensen (1981): Avian Dis., 25: 550-554.
Owens, G.M. and D.R. Ledoux (2000): Poultry Sci., 79 (Suppl 1): 113.
Panda, A.K., S.V. Ramarao, M.V. Raju and R.N. Chatterjee (2008): Br. Poult. Sci., 49: 592-599.
Patel, M.B. and J. McGinnis (1977): Poult. Sci., 56: 45-53.
Perry, R.W., G.N. Rowland and W.M. Britton (1991): Avian Dis., 35: 542-553.
Prinz, M., A. Hennig and H. Jeroch (1981): Arch. Tierernähr., 31: 519-526.
Robblee, A.R. and D.R. Clandinin (1970): Poult. Sci., 49: 976-981.
Robel, E.J. (1987): Comp. Biochem. Physiol. B, 86: 265-267.
Robel, E.J. (1991): Poult. Sci., 70: 1716-1722.
Robel, E.J. and V.L. Christensen (1991): Br. Poult. Sci., 32: 509-513.
Robel, E.J. (1992): Poult. Sci., 71: 1733-1738.
Robel, E.J., (1993): Poult. Sci., 72: 546-553.
Sallmann, H.-P., H. Fuhrmann and S. Götzke (1998): J. Anim. Physiol. a. Anim. Nutr., 80: 226-231.
Sell, J.L. (1996): Anim. Feed Sci. Technol., 60: 229-240.
Sell, J.L., M.F. Soto-Salanova, P. Palo and M. Jeffrey (1997): Poult. Sci., 76: 1405-1417.
Sell, J.L., D.W. Trampel and R.W. Griffith (1997): Poult. Sci., 76: 1682-1687.
Sheldon, B.W., P.A. Curtis, P.L. Dawson and P.R. Ferket (1997): Poult. Sci., 76: 634- 641.
Sklan, D., I. Bartov and S. Hurwitz (1982): J. Nutr., 112: 1394-1400.
Sklan, D., D. Melamed and A. Friedman (1995): Br. Poult. Sci., 36: 385-392.
Soares, J.H., J.M. Kerr and R.W. Gray (1995): Poult. Sci., 74: 1919-1934.
Soto-Salanova, M.F., J.L. Sell, E.G. Mallarino, F.J. Piquer, D.L. Barker, P.E. Palo and R.C. Ewan (1993): Poult. Sci., 72: 1184-1188.
Stevens, V.I., R. Blair and C. Riddell (1983): Poult. Sci., 62: 2073-2082.
Stevens, V.I., R. Blair, R.E. Salmon and J.P. Stevens (1984a): Poult. Sci., 63: 760-764.
Stevens, V.I., R. Blair and R.E. Salmon (1984b): Poult. Sci., 63: 1571-1585.
Stevens, V.I. and R.E. Salmon (1989). Br. Poult. Sci., 30: 877-887.
Sunde, M.L., C.M. Turk and H.F. DeLuca (1978): Science, 200: 1067-1069.
Surai, P.F., I.A. Ionov, E.F. Kuchmistova, R.C. Noble and B.K. Speake (1998): J. Sci. Food Agric., 76: 593-598.
Tripathy, S.B., S.G. Kenzy and W.J. Mathey (1967): Avian Dis., 11: 327-335.
Tuite, P.J. and R.E. Austic (1974): Poult. Sci., 53: 2125-2136.
Van Reen, R., M.W. Taylor and W.C. Russel (1951): J. Nutr., 43: 235-244.
Veltmann, J.R. Jr. and L.S. Jensen (1986): Poult. Sci., 65: 538-545.
Walser, M.M., G.F Hanlon, J.A. Newman, H.E. Dziuk, W.G Olson and P.E. Waibel (1980): Avian Dis., 24: 309-316.
Wen, J., P.A. Morrissey, D.J. Buckley and P.J. Sheehy (1996): Br. Poult. Sci., 37: 787- 795
Whitehead, C.C. and D.W. Bannister (1978): Br. J. Nutr., 39: 547-556.
Whitehead, C.C. and W.G. Siller (1983): Res. Vet. Sci., 34: 73-76.
Whiteside, C.H., T.M. Ferguson and J.R. Couch (1962): J. Nutr., 78: 162-166.
Whitmore, J.H., T.W. Sullivan and O.D. Grace (1968): Poult. Sci., 47: 159-164.
Zanboni, L., R. Rizzi and S. Cerolini (2006): Theriogenology, 65: 1813-1827.
Zhu, M., I.V. Wesley, R. Nannapaneni, M. Cox, A. Mendonca, M.G. Johnson and D.U. Ahn (2003): Poult. Sci., 82: 1559-1564.
Paper presented at the 2010 Multi-State Poultry Feeding & Nutrition Conference, held in Indianapolis, Indiana, USA (25 to 27 May 2010).
June 2010